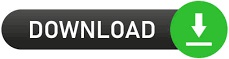
The presumptive mediolateral axis is indicated in the notochord and somitic mesoderm by curved lines (l, lateral m, medial). ( d) The vegetal view, comparable to ( a) shows the presumptive anterior–posterior axes in the notochord (dark grey) and somitic mesoderm (light grey) (curved arrows, a, anterior p, posterior). ( c) The MIB the cells become polarized with large filo-lamelliform protrusions on their medial and lateral ends (dark grey), which extend (black arrows), attach to adjacent cells or extracellular matrix and shorten (light grey arrows), thereby pulling the cells between one another along the mediolateral axis to from a narrower, longer array. ( b) The magnified view (the circled areas denoted by B in ( a)) of how cell intercalation produces convergent extension. The presumptive path of future shearing of the notochord with the somitic mesoderm is indicated (dotted grey arrow). Also, shown are the closure of the blastopore over the vegetal endoderm (straight arrows) and the site of convergent thickening (grey rectangles). ( a) The vegetal view of the IMZ of (i) the early Xenopus gastrula and (ii) the early neurula shows movements of involution (black curved arrows), convergence (short black, facing arrows) and cell intercalation (mediolateral intercalation behaviour, MIB grey fusiform shapes) in the presumptive notochord (dark grey) and somitic mesoderm (light grey). Understanding the patterning of these dynamic features of cell behaviour is important and will require analysis of signalling at much greater spatial and temporal resolution than that has been typical in the analysis of patterning tissue differentiation. It is not the nature of the cell behaviour alone, but the context, the biomechanical connectivity and spatial and temporal pattern of its expression that determine specificity of morphogenic output during gastrulation and blastopore closure. They are expressed progressively along presumptive lateral-medial and anterior-posterior axes of the body plan in highly ordered geometries of functional significance in the context of the biomechanics of blastopore closure, thereby accounting for the production of similar patterns of circumblastoporal forces.
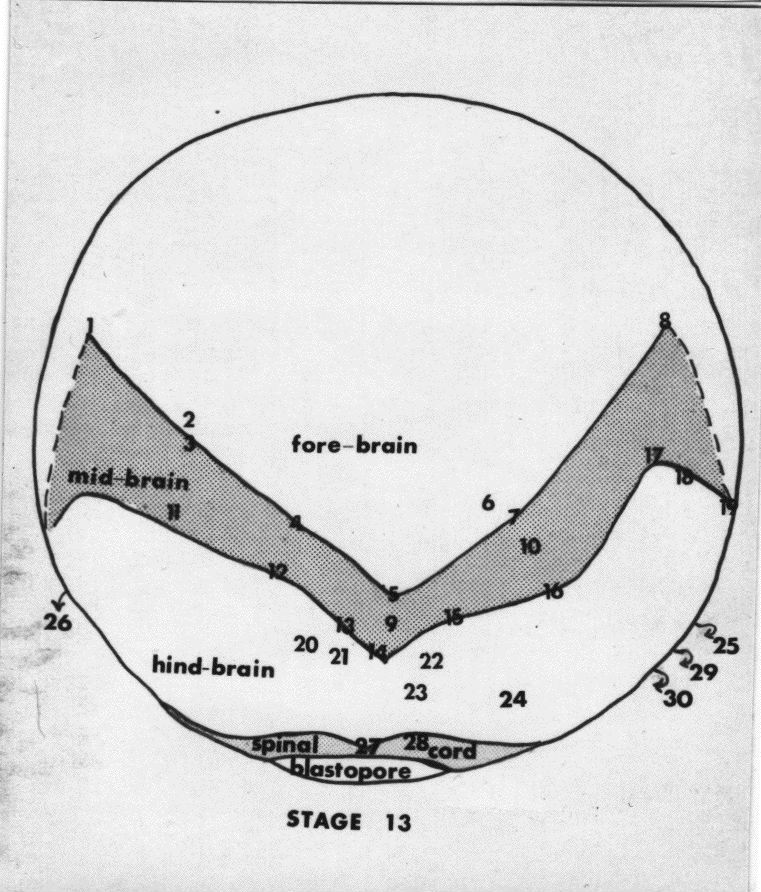
Although these cell behaviours are quite different and involve different germ layers and tissue organization, they are expressed in similar patterns. Mediolateral cell intercalation behaviour and epithelial-mesenchymal transition are used in different combinations in several species of amphibian to generate a conserved pattern of circumblastoporal hoop stresses. We review the dynamic patterns of cell behaviours in the marginal zone of amphibians with a focus on how the progressive nature and the geometry of these behaviours drive blastopore closure.
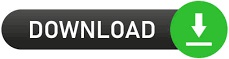